Research
Research
Summary
We are committed to broadly applying engineering mechanics to address prevalent challenges in cardiovascular biology, physiology, and medicine. We seek to understand the interactions between mechanics and cardiovascular disease, focusing on translating efforts and establishing disruptive technologies that advance patient patients. We leverage our expertise in solid and fluid mechanics, across the experimental and computational domains, to comprehensively character the mechanical stimuli vascular tissues are subjected to in the setting of health and disease. Our primary objectives are 1) to advance the diagnosis, prognosis, and treatment of cardiovascular disease in the clinical setting and 2) to understand how mechanics drive the structure, function, and remodeling of vascular tissues for targeted drug therapies. We enjoy collaborations with scientists, engineers, clinicians, and clinical-scientists.
We invite you to explore our research program below and publications.
Topics
Coronary Artery Disease Progression
Coronary artery disease (CAD) is the leading cause of death worldwide. A significant clinical challenge in the treatment of CAD is identifying patients at risk for rapid disease progression and potential rupture of an atherosclerotic plaque (i.e., plaque rupture). As mechanical loads have been postulated to play a central role in the development and progression of atherosclerosis, which is the underlying CAD, we seek to understand better the role of biomechanics in the natural history of coronary atherosclerosis in longitudinal clinical investigations. Research highlights include,
- demonstrating that distinct fluid-induced mechanical forces lead to increased plaque vulnerability and rupture potential
- identifying the interplay of solid and fluid mechanical loads on changes in atherosclerotic plaque size and composition
- establishment of novel computational approaches to determine the material properties of coronary tissues and characterize the 3D mechanical environment in patient-specific models of coronary arteries
- application of uncertainty quantification to understand the propagation of material parameter variability in the predicted coronary artery mechanical environment
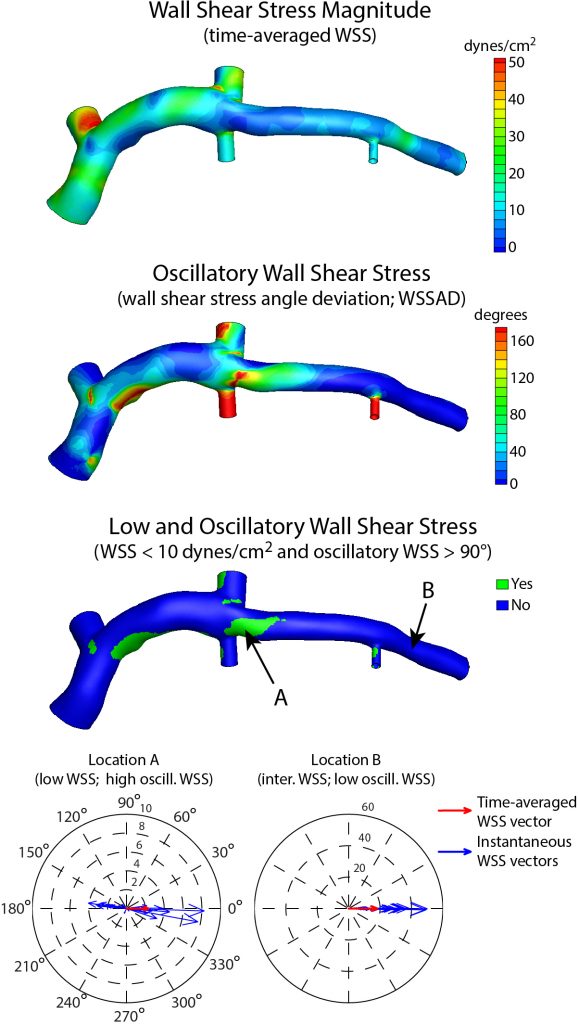
Timmins et al., J. R. Soc. Interface, 2017.
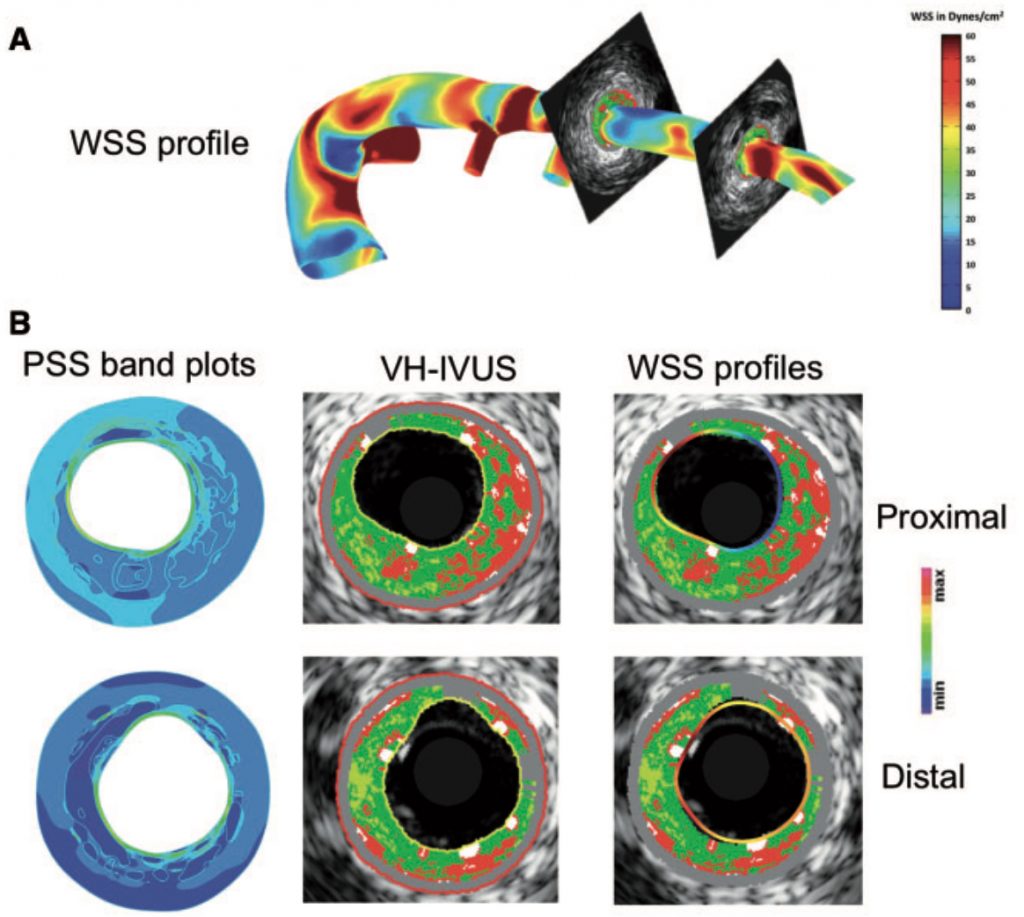
Costopoulus et al., Eur. Heart J., 2019
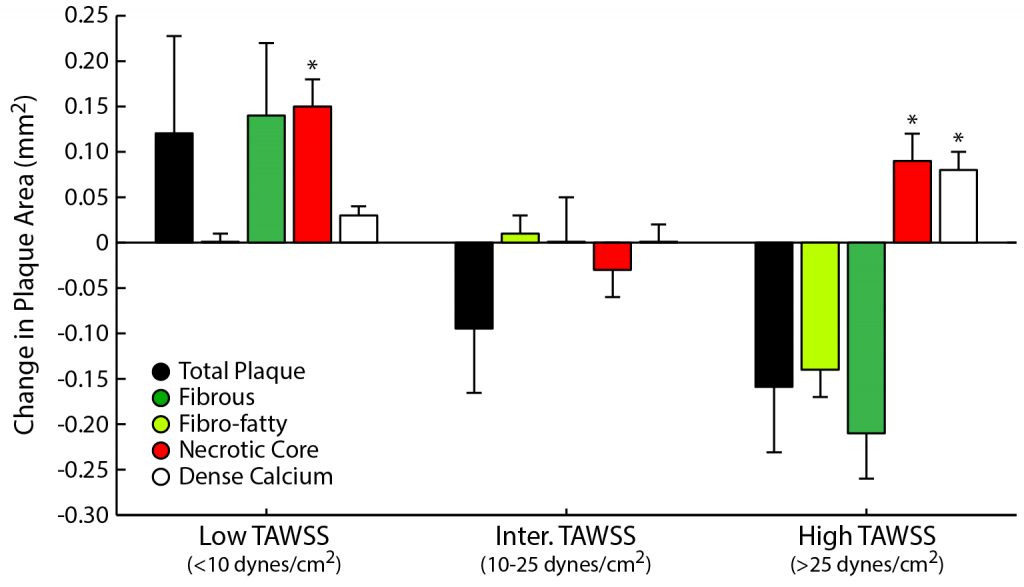
Samady et al., Circulation, 2011.
Stented Artery Biomechanics
Vascular stent implantation is one of the most common major medical procedures provided by the US health care system for the treatment of coronary heart disease (~600,000 stents implanted annually). Periprocedural complications following these interventions continue with failure rates as high as 20% depending on disease complexity and stent platform. A major focus of our research is directed at developing pre-interventional and post-procedural optimization strategies guided by biomechanics and intravascular imaging. Research highlights include,
- developing novel computational approaches to quantify the mechanical interaction between vascular stents and arterial tissues
- demonstrating that increased wall stress following stent implantation leads to a more aggressive pathobiologic response
- establishment of a deformable image registration framework to reconstruct the 3D deployed stent geometry through the fusion of multi-modal imaging
- development of a patient-specific, finite element framework to predict thein vivo stented artery mechanical environment
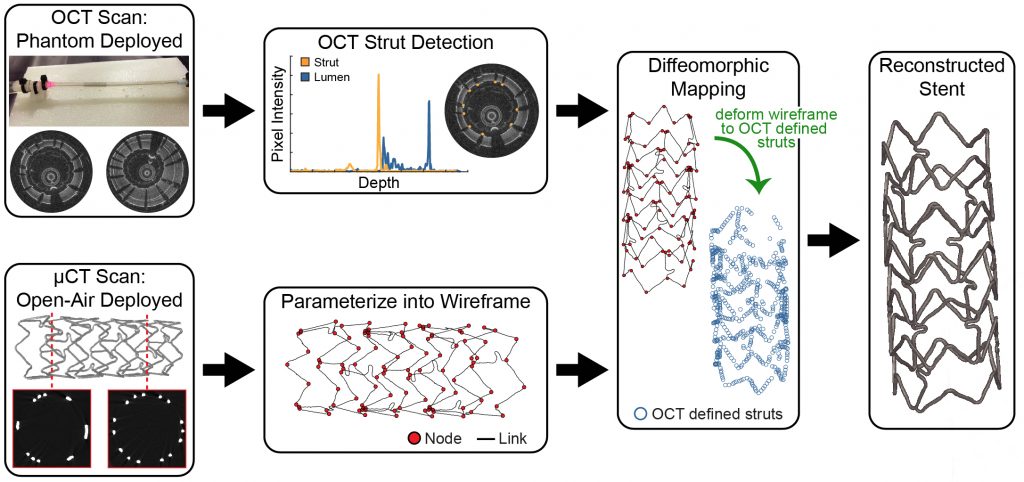
Elliott et al., IEEE Trans. Med. Imag., 2019
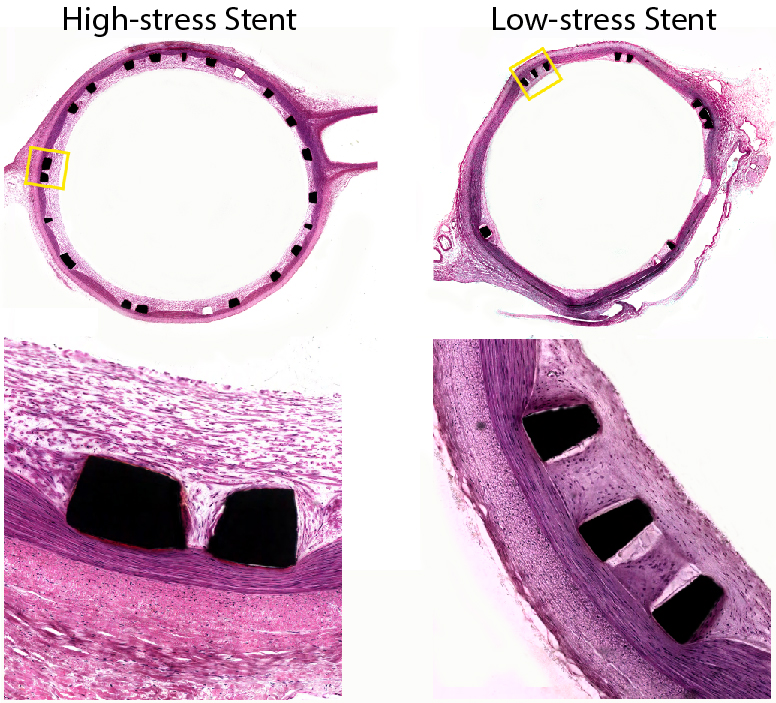
Timmins et al., Lab. Invest., 2011
Evaluation of In Vivo Hemodynamics with Cardiac Magnetic Resonance Imaging
Four-dimensional flow cardiac magnetic resonance imaging (4D flow cMRI) provides a non-invasive, non-ionizing radiation-based diagnostic and prognostic tool to directly interrogate the in vivo hemodynamic environment. My lab has pioneered efforts to utilize 4D flow cMRI to quantify patient-specific wall shear stress (WSS), which is a measure of the force flowing blood exerts on the endothelial cells that line blood vessels. WSS has been linked to the development and progression of numerous cardiovascular diseases and thus has great potential in the clinical management of patients across a range of cardiovascular pathologies. Areas of focus include:
- optimization of 4D flow cMRI acquisition, processing, and visualization strategies to advance the understanding of blood flow patterns
- development of a novel flow-based approach to quantify WSS directly from 4D flow
- demonstrating the improved accuracy of flow-based methods over velocity-based approaches to quantify WSS directly from 4D flow cMRI
- application of 4D flow cMRI to characterize the complex flow field in the setting of type B aortic dissection
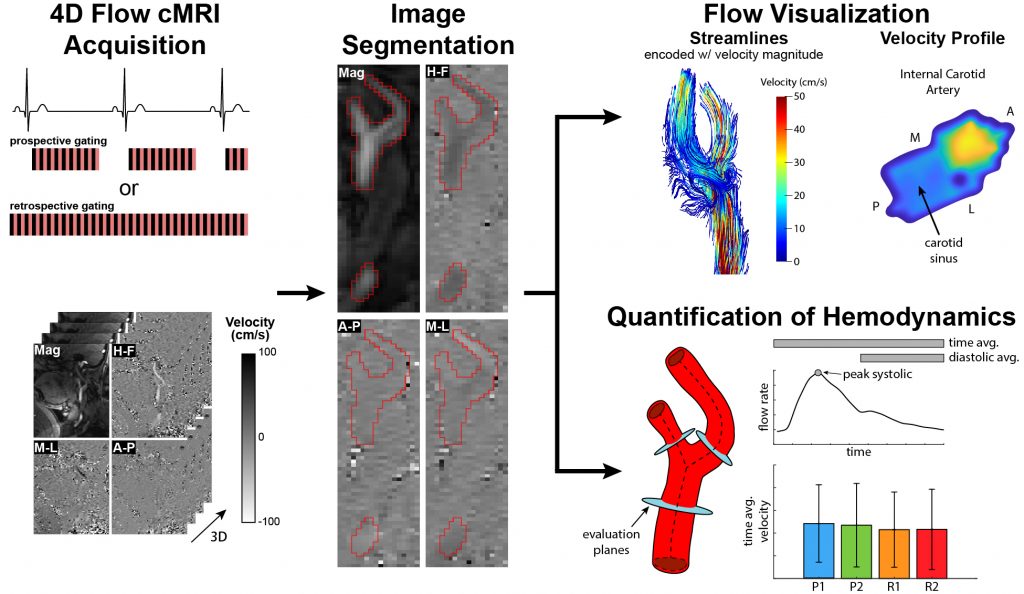
Hurd et al., Cardiovasc. Eng. Technol., 2023
Extracellular Matrix Organization, Remodeling, and Damage in Fibrous Tissues
The extracellular matrix (ECM) is a 3D scaffold comprised of collagen, elastin, proteoglycans, and other proteins and minerals that supports the surrounding cells in biologic tissue. Among other key functions, the biomechanical and biochemical properties of the ECM have implications for several pathologies, including cancer, fibrosis, and cardiovascular disease. We have sought to establish methods to evaluate the structure-function relationship, organization, and remodeling of the ECM in tissues where altered ECM dynamics are involved in disease development. Areas of focus include:
- application of nonlinear optical microscopy to identify structural inhomogeneity and fiber orientation in vascular tissue
- targeting ECM damage for therapeutic potential
- examining spatial and temporal changes in collagen and elastin organization with multiphoton imaging in a mouse model of atherosclerosis
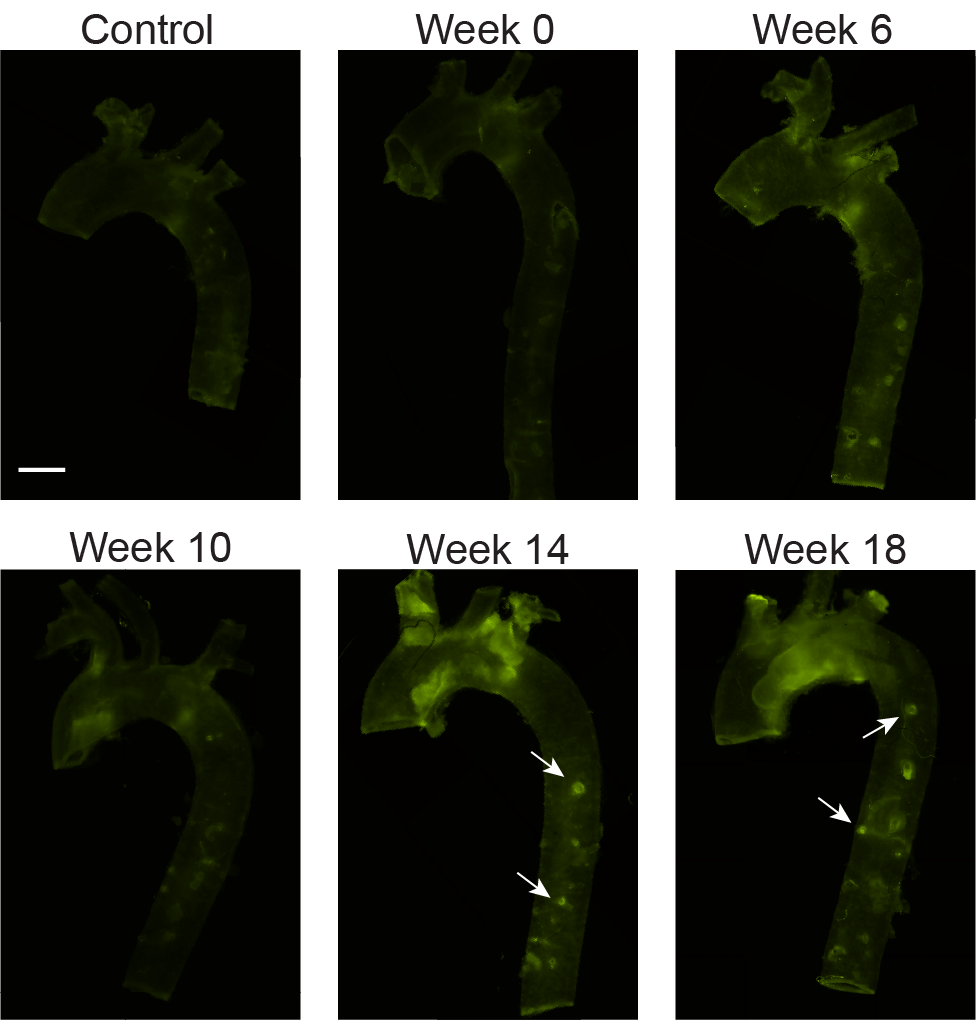
Smith et al., J. Cardiovasc. Trans. Res., 2023
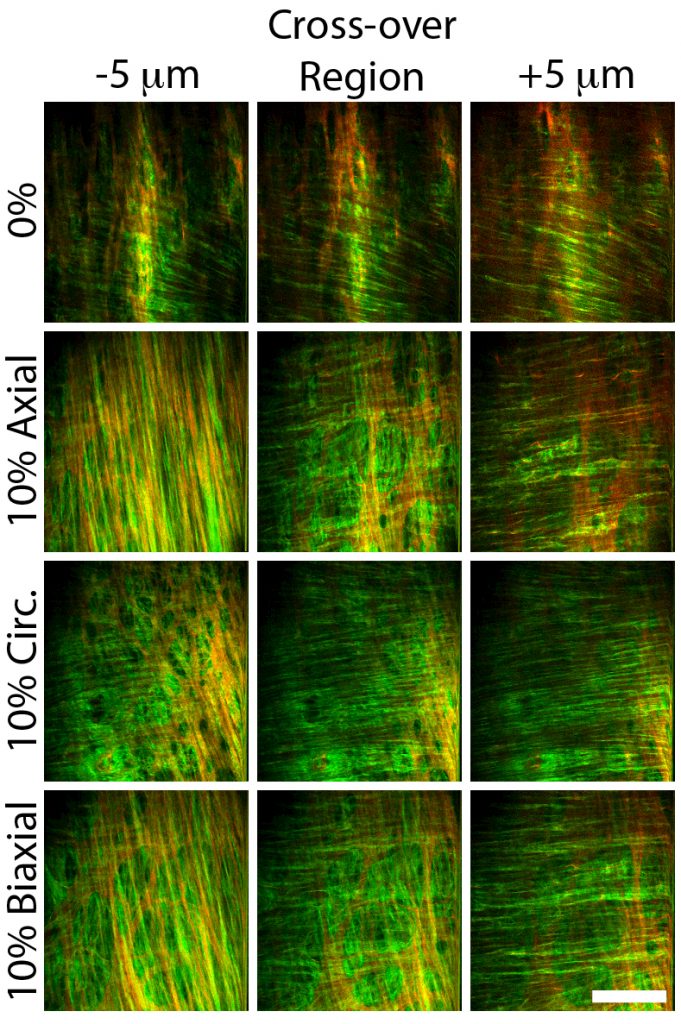
Timmins et al., AJP Heart and Circ. Physiol., 2011